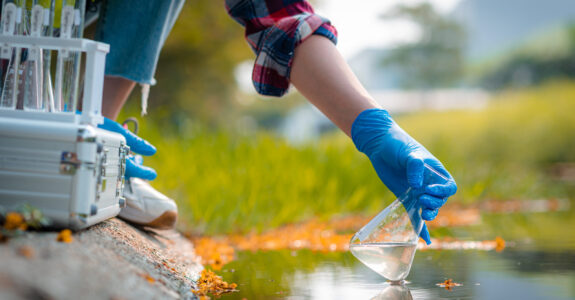
Understanding TFA: The Tiny PFAS with a Big Presence
December 17, 2024
By: David R. Blye, CEAC
We are surrounded by trifluoroacetic acid (TFA). This “forever chemical” linked to certain refrigerants, hydrofluorocarbons (HFCs), and fluoropolymers widely used in industries, is also present in consumer products like pesticides and pharmaceuticals. Wide-spread use has led to increased TFA concentrations in rain, soils, human serum, plants, plant-based food, and drinking water. New research is shedding light on TFA and other ultra-short-chain per- and polyfluoroalkyl substances (PFAS [C1 to C3]), revealing their alarming environmental persistence, pervasive presence, and questionable health risks across ecosystems. TFA has flown under the radar compared to its long-chain PFAS counterparts, but its unique characteristics have pushed it into the spotlight of scientific scrutiny.
What we know so far:
TFA is persistent.
TFA is known for its persistence due to its strong carbon-fluorine bonds, which make it chemically stable and resistant to degradation in natural environments. Additionally, TFA has a high solubility in water, allowing it to disperse widely in aquatic systems, frequently detected in rainwater, groundwater, and surface water. While short-chain PFAS like TFA are generally less bioaccumulative than their long-chain counterparts, concerns about the effects of chronic exposure remain due to its persistence. Recent findings published in Environmental Science & Technology reveal that TFA can bioaccumulate in various plants, presenting potential pathways for human exposure through the consumption of plant-based foods, juice, beer, and tea.
TFA is widespread.
TFA originates from a variety of sources, including being a breakdown product of certain refrigerants, HFCs, and fluoropolymers commonly used in various industries. Its global dispersion results from emissions from both industrial and consumer products, leading to TFA accumulation even in remote locations like polar regions and high-altitude ecosystems through atmospheric transport and precipitation. Notably, TFA is also produced naturally. According to Chemours Company (Chemours), when its refrigerant, HFO-1234YF, is released to the environment, it photo-oxidizes (degrades) and turns into TFA. Chemours also suggests that more than 95% of TFA naturally occurs from volcanic deep-sea vents, and over 200 million tons have been found in the world’s oceans.
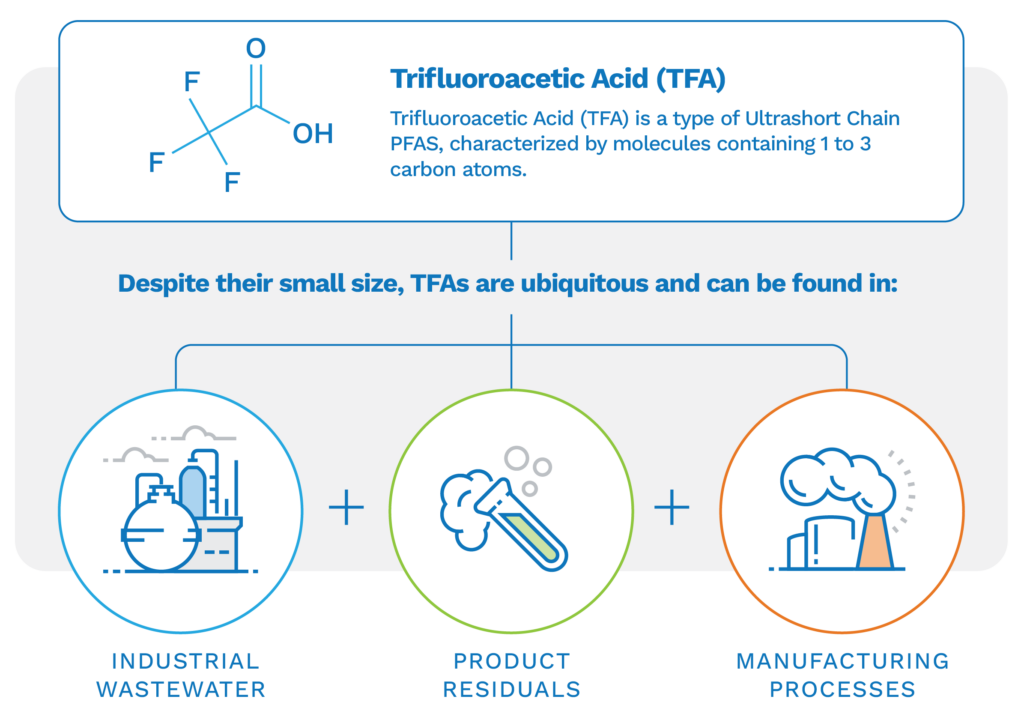
TFA brings questionable health impacts into play.
The long-term effects of TFA on human health and ecosystems remain largely unknown. Although TFA’s small size and high mobility reduce the likelihood of bioaccumulation, the health impacts are still not fully understood, and there is no consensus on potential toxicity. This raises concerns, given that longer-chain PFAS have been associated with various health issues, including fertility problems, liver damage, and cancer. Furthermore, TFA’s acidity and persistence may disrupt aquatic ecosystems and influence growth among plant and microbial populations.
TFA is tough to monitor, analyze, and regulate.
Like many PFAS, the TFA classification varies, with some definitions including TFA due to its single CF3, while others do not. Increasing regulatory interest is focused on whether the incineration of waste, particularly those containing PFAS or aqueous film forming foam (AFFF), effectively destroys PFAS while leaving TFA and other ultra-short-chain PFAS intact. Additionally, detecting, and quantifying TFA at trace levels in various matrices, such as water, air, and soil, poses analytical challenges, requiring more advanced techniques than those used by longer-chain PFAS, such as ultra high-performance liquid chromatography coupled with tandem mass spectrometry, known as LC/MS-MS. However, the ubiquitous nature of TFA causes elevated background concentrations in laboratories, as reflected in method bank analyses. This complicates detection by driving reporting limits to concentration levels well above the detection sensitivity of the instrumentation. Typical reporting limits in water for TFA are on the order of 50 to 100 ng/L, which typically represents a sensitivity above laboratory background. Most of the longer-chain PFAS are detectable to concentrations in the range of 2 – 5 ng/L.
Existing PFAS Treatments are Limited.
TFA is small molecule, extremely mobile and highly water soluble. The traditional water treatment technologies such as granular activated carbon (GAC) and ion exchange resins have demonstrated limited success for treatment/removal of TFA from the aqueous medium. However, ECT2 (a Montrose company) is evaluating new ion exchange resins that have demonstrated good removal efficiency. If it is determined that TFA is a health concern (human or ecological), treatment for this teeny, tiny PFAS is going to be challenging but differentiated solutions are available and exciting research is continuing to show improved treatment outcomes.
Key insights and actions needed to tackle TFA.
As we navigate the complexities of TFA and its implications, it is crucial for researchers, policymakers, and industry professionals to stay informed about its environmental persistence and potential health risks. Understanding the nuances of TFA requires expertise in analytical chemistry, environmental science, and toxicology to effectively monitor, assess, and manage this ubiquitous contaminant. As scientists and regulatory bodies work toward clearer guidelines for detecting TFA and developing effective treatment methods, it becomes increasingly important to take a proactive approach in assessing TFA levels in the environment by collaborating with the right partners who utilize the latest LC/MS-MS analytical techniques.
Interested in exploring TFA and its impact on your environment? Our laboratory environmental experts are here to help.
David R. Blye, CEAC
Senior Principal Chemist, Environmental Standards
Mr. Blye has more than 40 years of diversified experience in the field of environmental chemistry; his experience includes field data collection and environmental sampling and the planning, development, and execution of field sampling and analytical projects. He specializes in the interpretation of organic and inorganic analysis data and the development of field procedures for the collection of representative groundwater, surface water, soil, air, and multi-media samples. He has generated site-specific sampling plans for more than 200 sites, including Quality Assurance Project Plans for more than 47 state or federally supervised CERCLA, RCRA, and DOD sites. Mr. Blye has extensive experience in US EPA organic and inorganic analytical methodology and analytical data validation. He has overseen the validation efforts of several hundred projects. Mr. Blye also has extensive experience in auditing laboratory facilities to evaluate compliance with analytical protocols and Quality Assurance Project Plans and to determine analytical capabilities. He has audited more than 400 laboratories, including laboratories in Canada, the United Kingdom, Europe, and Puerto Rico. He also has a strong environmental forensics background and specializes in the analytical chemistry of PFAS, PCBs and Dioxins/Furans.